
Cryogenic cathodoluminescence (cryo-CL)
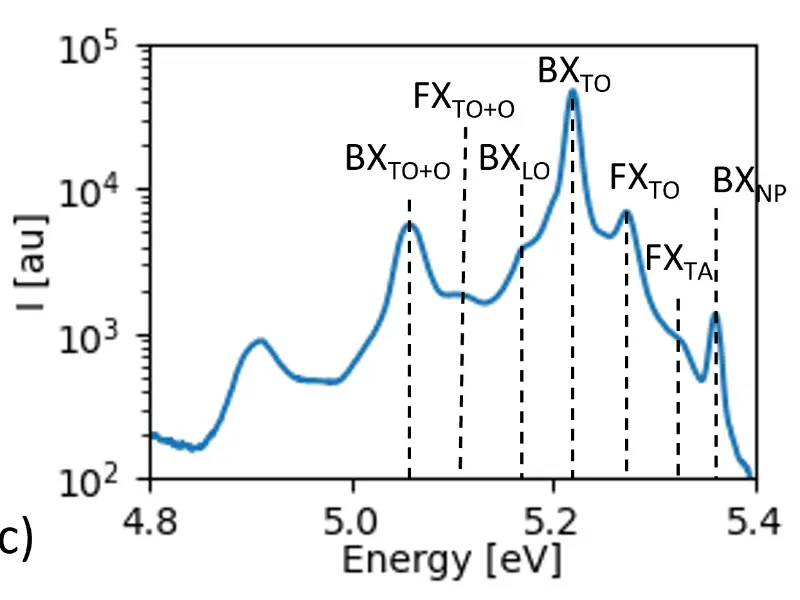
Introduction
Cryo-CL is cathodoluminescence performed on a sample cooled to cryogenic temperatures, typically liquid nitrogen temperatures or below. Reducing the thermal agitation in the sample increases the luminescence intensity, enables the discrimination of narrow peaks within broad emission bands, and stabilizes exotic quasiparticles called excitons. Cryo-CL is thus most often used for samples which exhibit weak luminescence at room temperature, or which have fine spectroscopic features that are close in energy and therefore difficult to resolve.
Principles
Performing CL experiments at low temperature significantly impacts the obtained spectra. The main effects and mechanisms involved are discussed below.
Sharper spectral features
At low temperatures, spectral features in luminescence signals tend to become sharper as two distinct broadening mechanisms are reduced:
- Electronic thermal broadening
Thermal agitation at room temperature broadens the energy distribution of carriers (Figure 1) in a semiconductor. This in turn broadens the luminescence peaks involving a band edge as the carriers may recombine from a broad range of states. At cryogenic temperatures, the distribution of carriers become narrower as the Fermi-Dirac distribution approaches a step function.
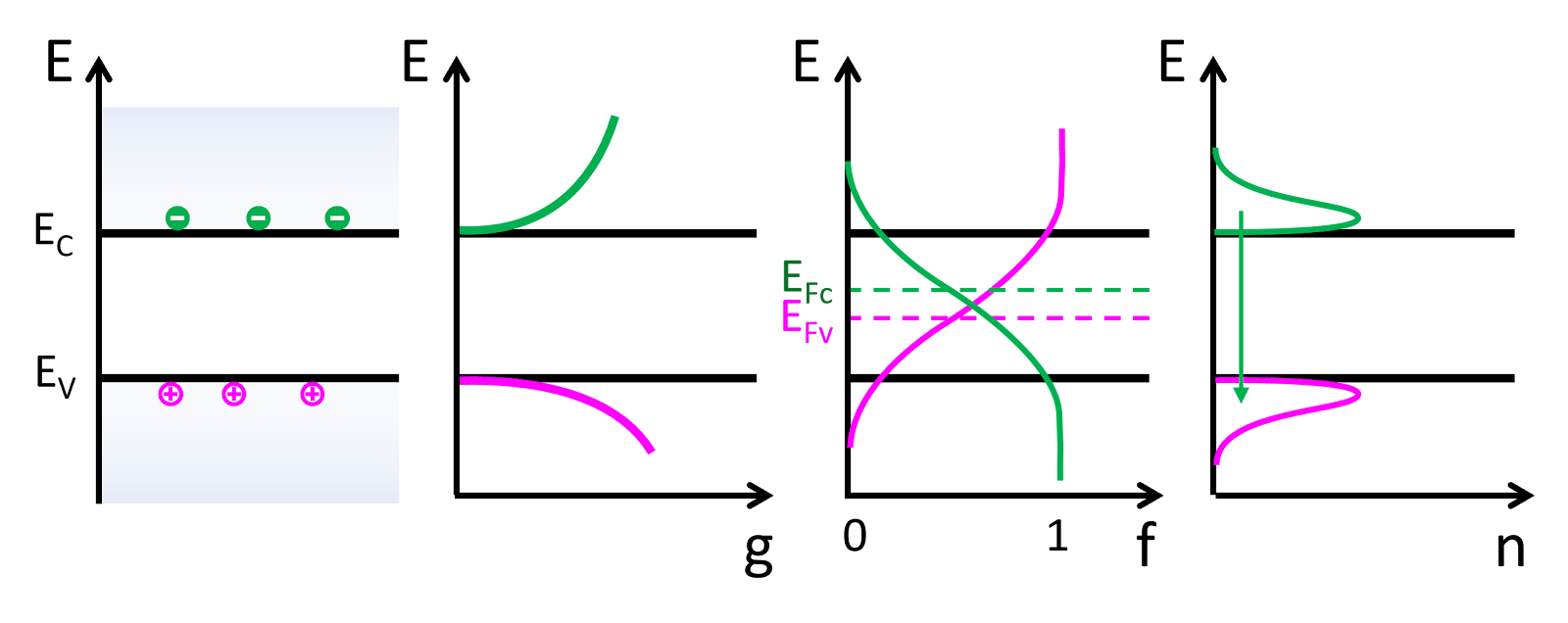
- Phonon broadening
Electron-phonon interactions lead to peak broadening. Phonon broadening is reduced at cryogenic temperatures as the vibrational modes are less active.
It is noteworthy that cooling the sample does not reduce the width of all spectral features because of residual phonon broadening and other broadening mechanisms such as:
- Inhomogeneous broadening:
The presence of impurity, defect, alloy, or strain inhomogeneities in the material leads to variations in local emission energy and to a broadened overall peak.
- Density-dependent broadening:
At higher densities, carriers can interact with each other, leading to broadening due to the spread in interaction energies.
Stronger luminescence signal
Lower temperatures reduce carrier-phonon interactions, which are responsible for non-radiative recombination processes. This increases the likelihood of radiative recombination, resulting in a stronger luminescence signal.
Higher exciton stability
After excitation, the electron and hole created in proximity attract each other and may combine in a hydrogen-like quasi-particle called an exciton. Free excitons move freely within the crystal. A free exciton may bind to a localized lattice defect or dopant, forming a bound exciton. When an electron and a hole that are paired within an exciton recombine, the recombination energy is reduced by the exciton binding energy. Consequently, excitons emit at energies a little below the band gap.
Free excitons whose binding energy is small compared to kT dissociate into free carriers. Likewise, weakly-bound excitons dissociate from their defect to become free excitons. As kT is reduced, excitons become more stable.
Instrumentation
The sample is typically cooled down to near liquid helium or liquid nitrogen temperature using a continuous-flow cryostat (Figure 2). The sample temperature is usually regulated using a resistive heater and a PID control loop.
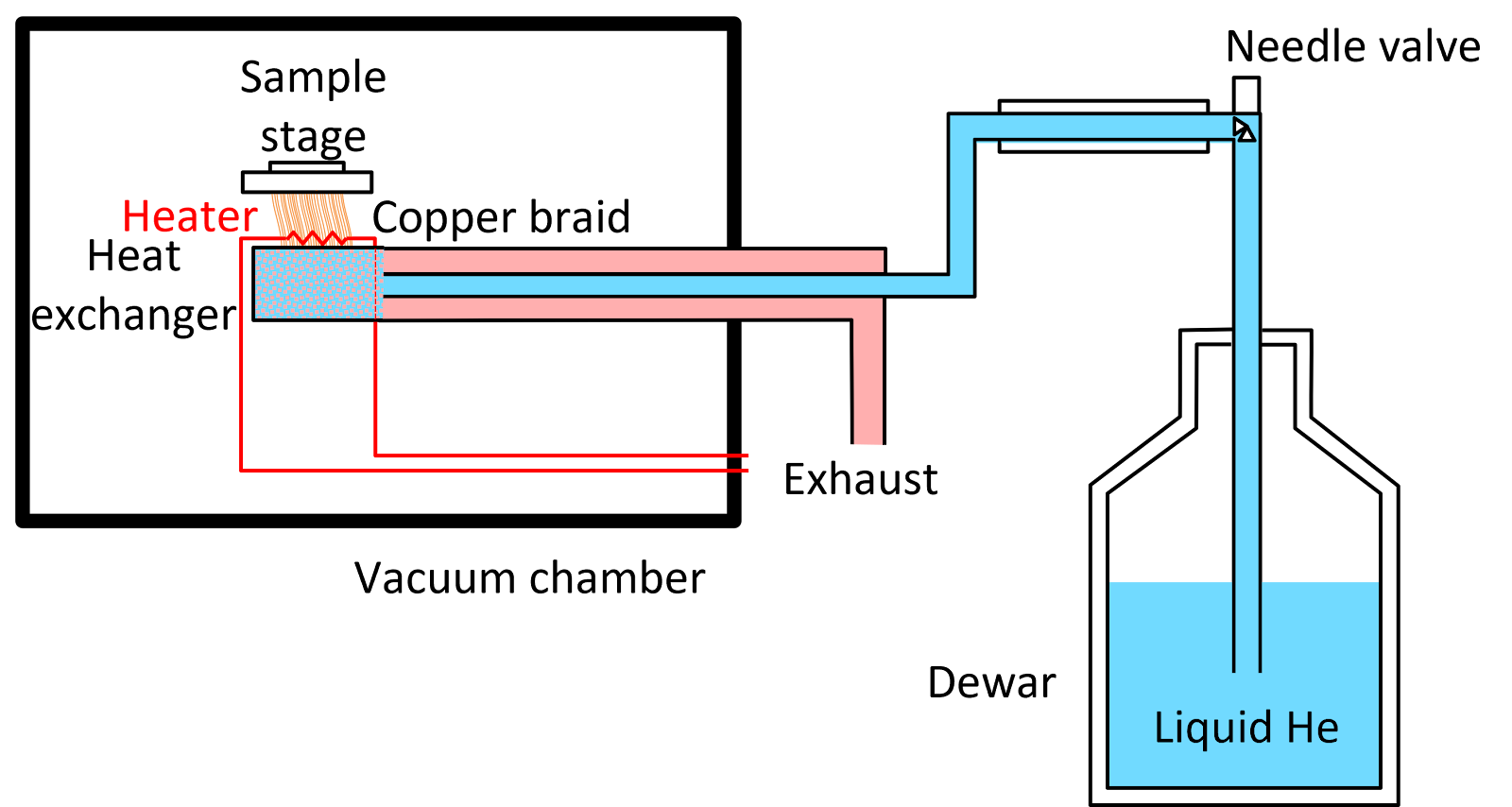
Example results
- The reduced thermal peak broadening at low temperature enables the discrimination of spectral features in GaN that are hardly visible at room temperature (Figure 3a).
- The enhanced luminescence signal at low temperature enables the detection of the weak emission of Si, an indirect bandgap semiconductor (Figure 2b). Peak attribution comes from Reference [1].
- The enhanced exciton stability enables the detection of the emission peaks from free excitons (FX) and bound excitons (BX) in diamond, as well as their phonon replica (Figure 2c) [2].
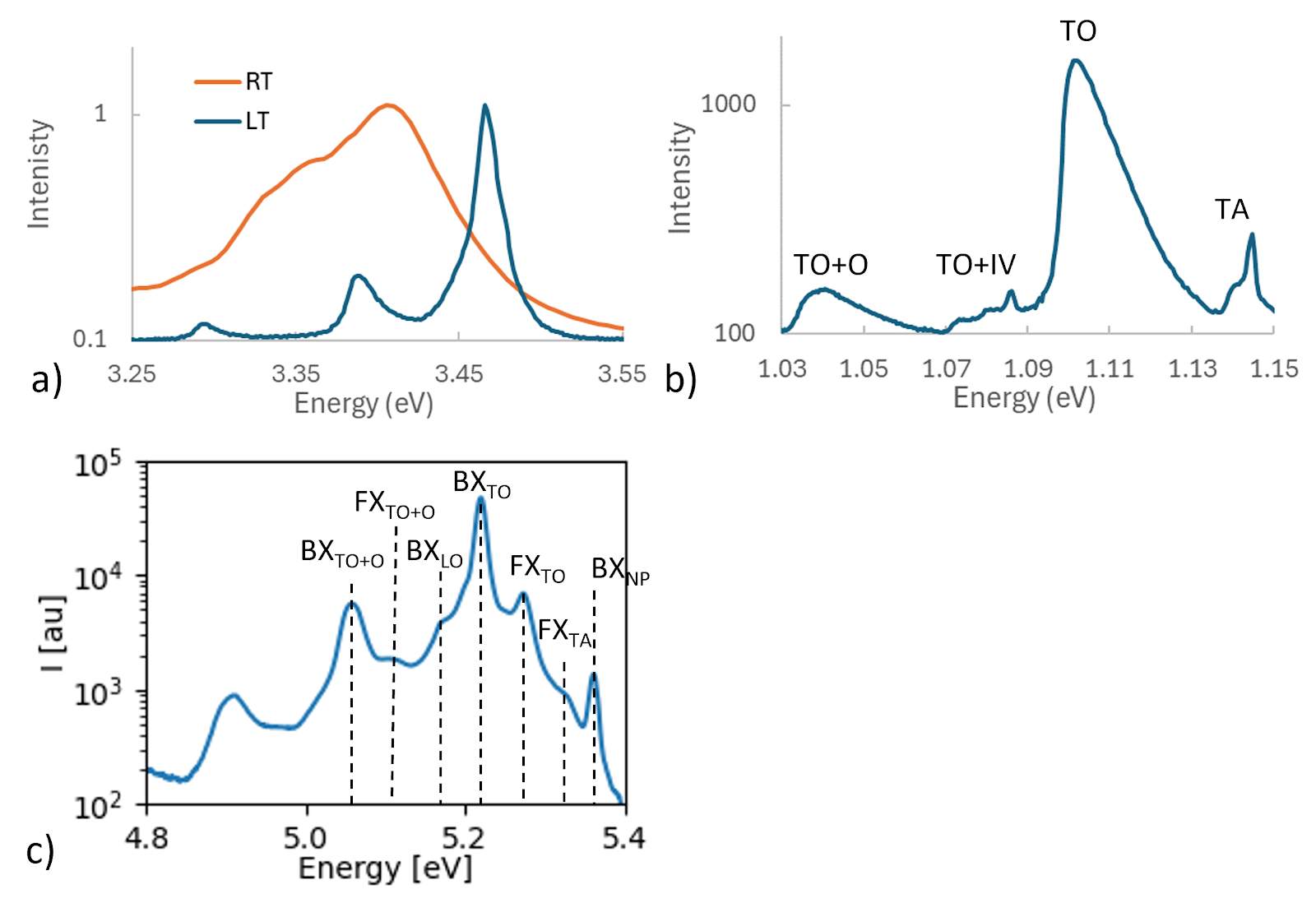
Benefits and Further Reading
Benefits and Further Reading
- Enhanced luminescence for weakly-emitting samples
- Sharper spectral features
- Stabilization of both free and bound excitonic states
[1] P.J. Dean, J.R. Haynes, and W.F. Flood, Phys. Rev. 161, p. 711 (1967).
[2] N. Tappy, P. Gallo, A. Fontcuberta i Morral, C. Monachon, Carbon 191, p. 48 (2022).
Latest Scientific Publications
Explore our high-resolution cathodoluminescence tools designed for superior materials characterization.